Communications
Radio waves can convey information in the form of sounds, pictures, and data. We can confine the waves within a coaxial cable transmission line, e.g. in cable TV, or transmit them through space using antennae for wireless communications. Wireless communications is ubiquitous today, for broadcast radio and TV, personal communications via cell phones, GPS navigation, and interconnecting electronic devices using Wi-Fi and Bluetooth. Communications applications are so important that many learned societies focus on it. In contrast, IMPI focuses on non-communications applications.
Radar
Radar determines the position and velocity of some object, e.g. an aircraft or land vehicle, by bouncing an electromagnetic wave off the object. The radar set consists of a transmitter that emits a short pulse of electromagnetic waves usually with a directional antenna, and a receiver connected to the same antenna. The direction to the object is determined by the direction in which the antenna is pointing when a reflected pulse is detected. The distance to the object is determined by the time between when the pulse is transmitted from the radar antenna, and when the reflected pulse is received. The velocity of the object towards or away from the radar set is determined by measuring the frequency of the reflected wave. Because of the Doppler Effect, if the object is moving towards the radar set, the frequency of the reflected wave is increased in proportion to the velocity, and the opposite if the object is moving away from the radar set.
Heating & Cooking Food
The Microwave Oven
Heating food in domestic microwave ovens is the best-known application of microwaves. Approximately 80 million microwave ovens are produced per year. Ovens vary from simple inexpensive counter-top units, to built-in units that often incorporate multiple cooking technologies (e.g. infra-red radiation and convection). While most consumers mostly use their “microwave” for simple tasks like heating beverages, prepared foods (e.g. pizza), and leftovers, the microwave oven can be used to cook complete meals from raw ingredients.
In other forms of cooking, heat is absorbed at the surface of the food, and must diffuse inward so that the entire volume of the food is cooked, and this thermal diffusion process is slow. In contrast, microwaves penetrate into the food, and thus heat the food in depth, considerably reducing the cooking time. This translates into healthier food. Exposure to high temperature for long times destroys nutrients and natural flavors. Furthermore, cooking in water can leach nutrients from the food into the water. The nutrients cannot be replaced and most cooks add salt and oil-rich sauces to replace lost flavor. Because microwave cooking is faster, and uses no or less water, more of the nutrients and natural food flavors are preserved and less salt and sauces are added.
Click here for some recipes from the Microwave Technologies Association.
Click here for some more recipes from Marie Jirsa.
Click here for some more recipes from Ray Boxman


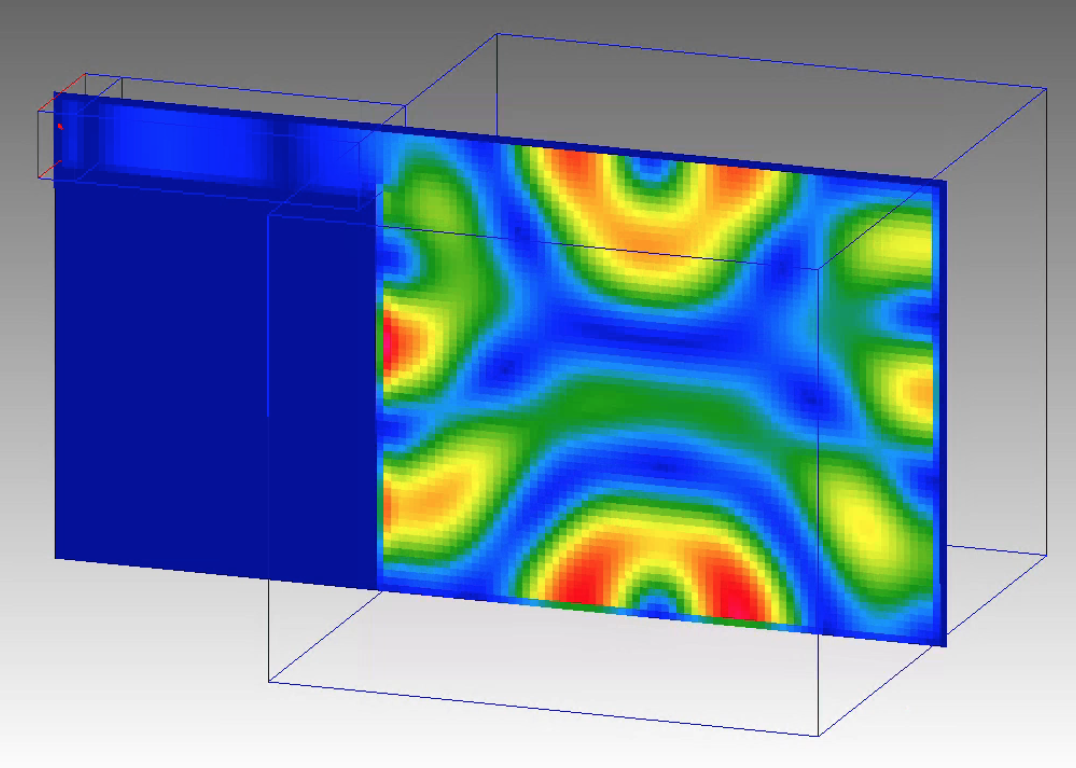
Microwaving Frozen Meals
Frozen prepared meals are very popular. While the most frequent use of domestic microwave ovens is re-heating leftovers, heating microwaveable food products ranks second [IMPI Survey 2018]. Frozen meals are classified as either “ready to eat” or “not ready to eat”. Most frozen prepared meals are ‘not ready to eat’. This means they need to be heated to a safe temperature (usually 160 or 165 ∞F) to kill pathogens, in the unlikely event that they are present. It is important to carefully follow the manufacturer’s cooking instructions, and to use a digital food thermometer with a thin tip to probe several areas of the dish and verify that the safe temperature has been reached everywhere.
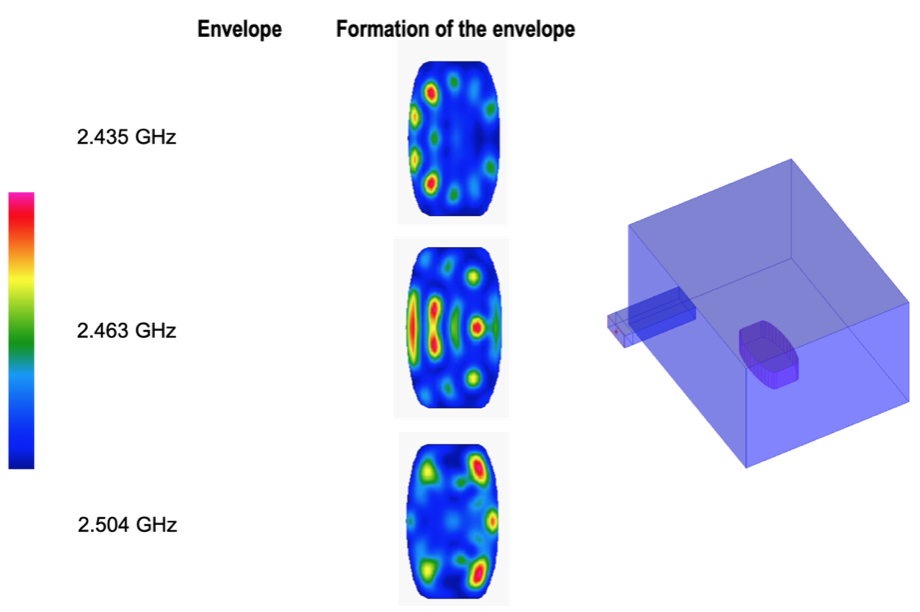
To evenly heat to safe temperatures, many dishes require stirring at some time. This is necessary, because the product – contrary to popular belief – does not heat from inside out. Instead, the edges of the food tray tend to absorb microwaves better than the center, which makes them defrost earlier. Then, another effect kicks in: Defrosted food absorbs microwaves faster than frozen food. As a result, the edges of a frozen food tray may already boil, while the center is still frozen. Stirring alleviates this problem, because it evens out the temperature everywhere in the tray. Another strategy is to use a lower microwave power setting, so that the temperature gets more time to equilibrate. Using twice the power to heat a frozen dish does not mean you can do it in half the time. In many cases, the results with lower power are much better, and the process is not much slower. Frozen meals are often enclosed in a thin polymer film. This film plays an important role: Leaving the film on captures steam generated from the food. This steam carries some of the heat to areas that would otherwise stay cold. The infrared images below show how stirring and retaining the film produce a more evenly heated meal.
Temperature equilibration also happens after cooking. There may be some colder areas left somewhere in the tray, which receive heat from the surrounding hotter areas. This explains another popular belief, namely that microwave-heated food cools down faster.
Many microwaveable meals have dual instructions, meaning that they may be heated in a conventional or in a microwave oven. For larger portions, such as family size lasagna, even combined instructions are sometimes available. In these cases, the consumer can save time by using the microwave oven, while the conventional oven is pre-heating. The second phase of cooking then takes place in the conventional oven where browning and crisping occur.
Industrial Food Processing
Engineers have developed a wide range of microwave heating technologies that help the food industry reduce preparation time, increase energy efficiency, reduce waste, and ensure microbial safety of the food products. Their products are distributed through the food supply chain for use in restaurants and for sale in grocery stores. For example, microwave systems in food processing plants pre-cook bacon used to make sandwiches in fast-food chain restaurants. This reduces meal preparation time in the restaurants, and the fat rendered from the cooked bacon in the food plants is collected as an ingredient for other products. Microwave systems are also used in food plants to temper (bringing the temperature from deep freezing to just below the freezing point of water) large blocks of frozen meat before grinding to make hamburger patties sold in grocery stores. Volumetric microwave heating shortens the tempering time of over 12 hours in chilled rooms to a few minutes.
Foods are not only sources of nutrients and energy for humans — they can also support the growth of micro-organisms. New technologies based on microwave heating have been developed for the food industry to control foodborne microbial pathogens in pre-packaged foods or ready-to-eat meals. The rapid internal heating of the packaged foods in microwave systems inactivate the target micro-organisms while retaining sensory quality and nutrients in the food products. Microwave pasteurization is used by the food industry to kill bacteria that can cause disease (e.g. Salmonella, Listeria and Campylobacter) as well as fungi and other microbes that can degrade food quality.
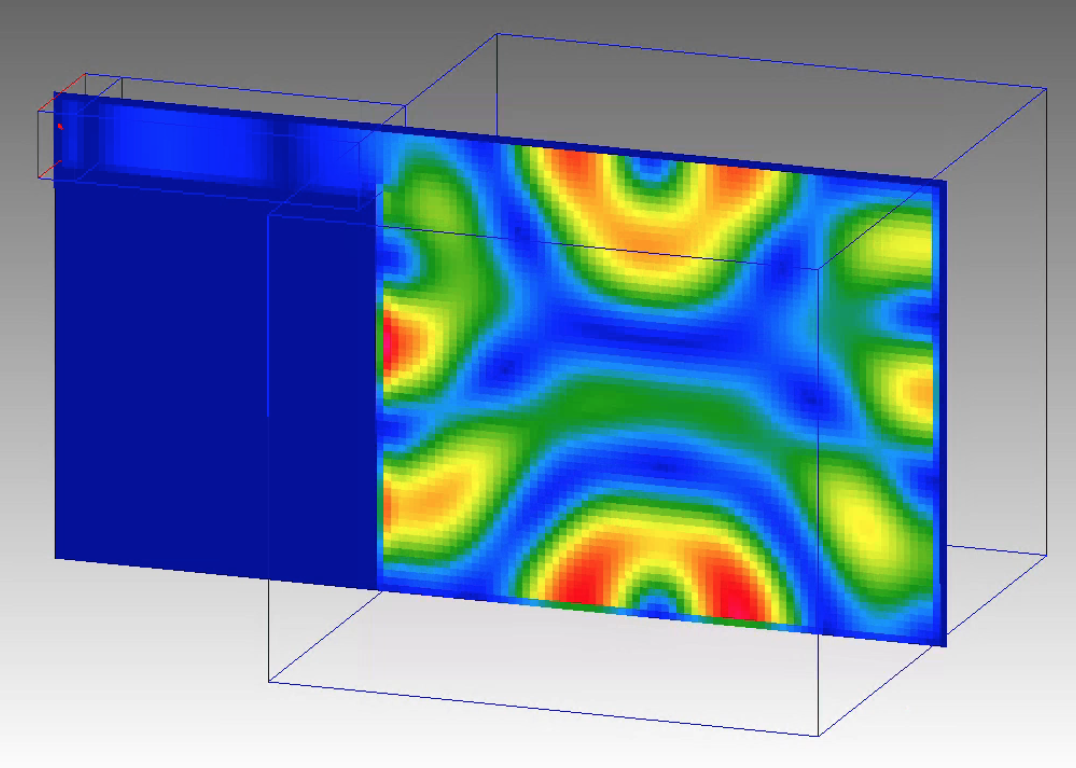
For example, the new microwave assisted thermal sterilization (MATS) technology developed at Washington State University is now used in commercial production of shelf-stable ready-to-eat meals for retail markets in India. A continuous process line is able to produce over 30 single meals per minutes.
Another microwave process, developed at Texas Tech University, does not affect taste or texture, and usually can be applied in the final packaging, just before shipment. Using such technologies saves the food industry money and contribute to a green planet by reducing food waste.
Untreated tortilla, after 17 days on the shelf, is covered with mold (left). Identical tortilla, treated with Micro-Zap process, after 17 days on the shelf. (courtesy of Brian Blackwell) (Right).
Chemistry
Microwaves are used for organic/polymer synthesis, medicinal/drug chemistry, material science, heterogeneous catalysis, nanotechnology, biochemical processes, and energy conversion processes (e.g. pyrolysis and gasification). Often microwave-assisted reactions have higher reaction rates, and in many cases microwave heated reactions can generate products that cannot be generated by conventionally heated chemical reactions at the same temperature. This is because the microwave energy is absorbed throughout the volume of the material (rather than only at the surface), so that the heating rate can be much higher than with other heating techniques. Furthermore, when heating mixtures of materials, sometimes the microwave energy will be absorbed faster in one selected material than in the others.
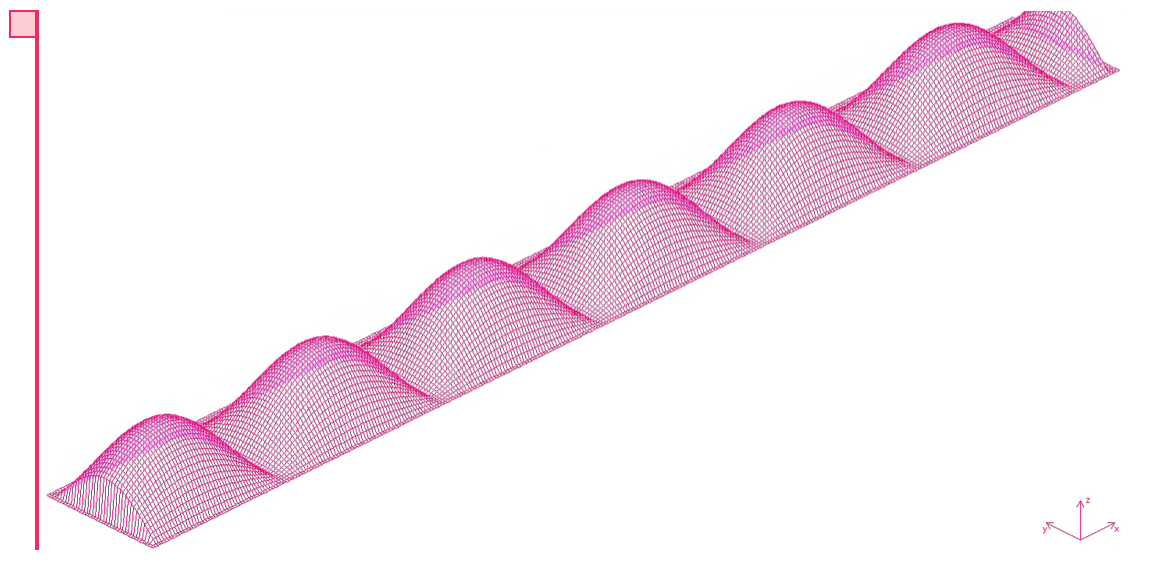
Microwave chemistry can be divided into two general categories: (1) liquid phase (solvent-based) chemistry and (2) solid-gas phase chemistry. In solvent-based chemical reactions, microwaves can deliver heat to the reaction in several ways. Polar solvents (e.g. methanol, acetonitrile, diethyl ether) are heated directly by dipole rotation (similar to water, described earlier), and solvents with high ion concentration (e.g. ionic liquids, deep eutectic solvents) generate heat by vibration of ions with the alternating microwave field. For non-polar solvents (e.g. hexane) that cannot be heated directly by microwave energy, a material which absorbs microwave energy and heats the surrounding material, called a susceptor, is added.
Microwave heating of systems containing both solids and gases is more complicated. Microwave heating of solid materials can be particularly complex if the material is not uniform. Many useful solid materials are comprised of microscopic regions, which differ in composition and structure, called phases. Each phase may interact with the microwaves differently. The different phases may absorb microwave energy through different mechanisms, e.g. dipole rotation (as described earlier for water), ion conduction, and dielectric losses at the interface between two different dielectric materials. Combinations of these mechanisms may occur simultaneously when different phases are present in the same material. For example, Solid catalysts may have a support phase, an active phase, and air domains, which interact differently with microwave fields based on their dielectric properties, and can result in non-homogenous heating within the material. Localized hot spots can occur in phases within the material that are heated most by the microwave field. These hotspots can be used advantageously by targeting heating of certain phases of a catalyst to accelerate reaction rates on active catalyst sites. This selective heating can selectively generate products while maintaining lower bulk catalyst temperatures. Lower temperatures translate to using less energy and lower costs.
Material science research aims to engineer novel catalytic materials that maximize the benefits of selective microwave heating for particular reactions. This allows targeting specific active sites in the material for heating while keeping the inactive sites relatively cooler. This increases the reaction rates at the targeted sites and enables increased catalytic process efficiencies.
Many different types of products can be produced using microwave-assisted chemistry. Microwaves speed up synthesis of organic molecules, pharmaceutical drugs, and nanomaterials. They are used in heterogeneous catalysis in many applications including conversion of methane into hydrogen and valuable chemicals, desulfurization of petroleum, catalytic cracking of bitumen, and conversion of waste plastic materials into their monomeric building blocks. Microwaves regenerate environmental catalysts used to remove pollutants from the environment (e.g. CO2, NOx, SOx, particulate matter, and volatile organic compounds). In biochemistry, microwaves synthesize peptide chains and speed enzyme digestion of proteins. For energy conversion, microwaves are used in pyrolysis and gasification of solid fuels for generation of synthetic natural gas with coproducts of tars and carbon residues.
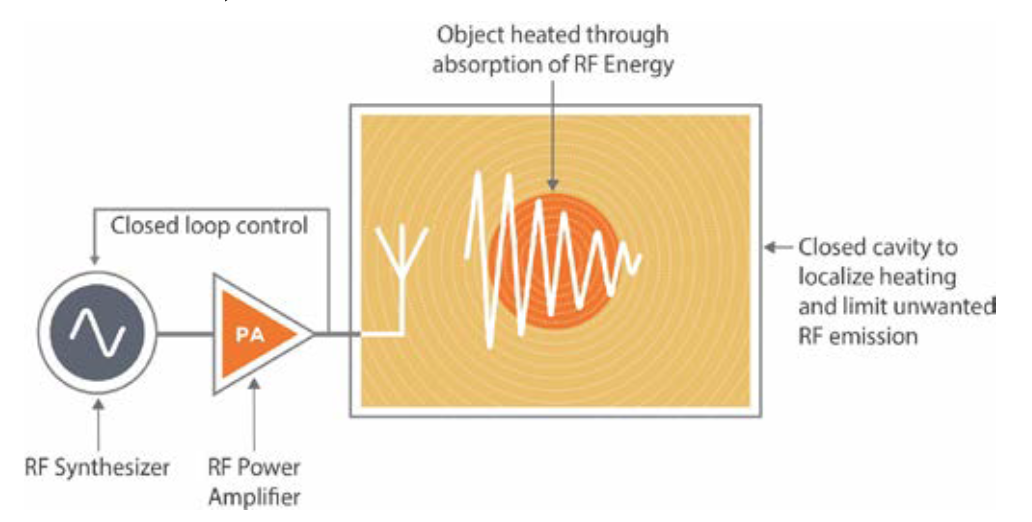
Material Processing
Microwave drying is commercially used for drying food products, agricultural products, and solid fuels (e.g. coal, biomass). Microwave drying reduces drying times compared to conventional thermal drying processes. Microwaves selectively and rapidly heat polar water molecules within the wet material, and eventually evaporate them. Dissolved salts in the water can increase the microwave heating ability and, in turn, increase the drying rate. These salts break down into charged ions that vibrate in the alternating electromagnetic field and heat the solution when they collide with molecules in the solution.
Microwave sintering is a process in which ceramic powders are densified at very high temperatures to form solid ceramic materials. Microwave sintering improves the densified ceramic material characteristics (refined microstructure and mechanical properties) compared to those generated by traditional thermal sintering techniques. Furthermore, traditional techniques require high pressures to densify the powders whereas microwaves are able to sinter materials at ambient pressure, thus increasing efficiency and reducing cost.
Silicon carbide (SiC) fiber “tows,” used as reinforcements in ceramic matrix composites (CMC) for aircraft and rocket engine parts that operate in harsh, high-temperature environments, processed with low-cost microwave sintering to tailor the strength of the tows and heal damaged fibers. (Photo Credit: Jordan Salkin. GRC-2019-C-05624 | Silicon carbide (SiC) fiber “tows,” which… | Flickr CC BY 2.0)
Microwave and RF energy has been applied to various other material processing techniques such as graphene formation, synthesis of quantum dots, chemical vapor deposition (e.g. diamond films and growth), rubber vulcanization, polymer curing, metal brazing and joining, water treatment, soil decontamination, and biodiesel production.
The reactor chamber of laboratory-scale Hybrid Physical-Chemical Vapor Deposition (HPCVD) system in the Pennsylvania State University, US. A stainless steel susceptor sits on a quartz rod inside the water-cooled chamber. During deposition, it will be heated by an inductive heating coil (copper tubing outside the chamber). A silicon carbide (SiC) substrate and magnesium pellets are on the top of the susceptor. (source: Wikipedia)
Medicine
Radiothermy, short wave diathermy, and microwave diathermy are terms used to describe the application of electromagnetic waves to some portion of the body, in order to warm it a few degrees. This encourages blood flow, which accelerates healing of injuries and can relieve pain.
The use of higher dosages of electromagnetic waves to the extent that selected tissues are damaged or destroyed is termed variously diathermia induced hyperthermia, electro-surgery, electrocautery, and RF ablation, depending upon where and how they are applied. For example, RF ablation in which an antenna is inserted via a catheter to the heart (and hence often called catheter ablation) is used to destroy selected heart tissue that causes arrhythmia (uneven or fast heartbeat).
Agriculture
Electromagnetic waves are used in the agricultural and forestry industries. Green timber is pre-treated to create micro-steam-explosions in the wood cells. This increases wood drying rates over conventional systems by 3 to 5 times and allows better uptake of preservatives and polymers to create more durable timber products. Grains are treated prior to storage and shipment to kill pest and pathogen infestations for better biosecurity. Animal feeds are treated to improve feed digestibility by 10 % or more. Soil is pasteurized to kill weed seeds and soil borne pathogens, increasing crop yields by up to 30 % under field conditions. Seeds and young crop plants are irradiated with low dosages, to accelerate their germination and improve their early vigor, growth rate and productivity. Hypothermic animals and crop plants are warmed to save their lives during cold snaps. Precious oils and pharmaceuticals are extracted from plant materials without using harsh chemical processes. Valuable gas, oil, and biochar are recovered from the almost 40 % of food that is spoiled or wasted around the world, through microwave assisted pyrolysis. In most cases, adopting electromagnetic processing significantly reduces energy requirements, compared with more conventional heat sources, and in some cases achieves outcomes that are not possible from conventional systems because of the very rapid heating or because of the energy available directly from the electromagnetic wave itself.
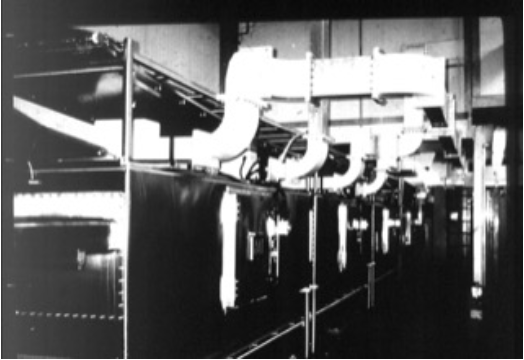
Plasma
Plasma, the 4th state of matter, is produced industrially using an electrical discharge, i.e. passing electrical current through a gas. While d.c. current may be used, there are advantages is using an RF or microwave discharge: often the plasma production is more efficient, can be better controlled, and better uniformity can be obtained. RF and microwave plasmas are used for several purposes including lighting, cleaning surfaces (particularly in the semiconductor industry), and depositing thin films (e.g. of diamond).
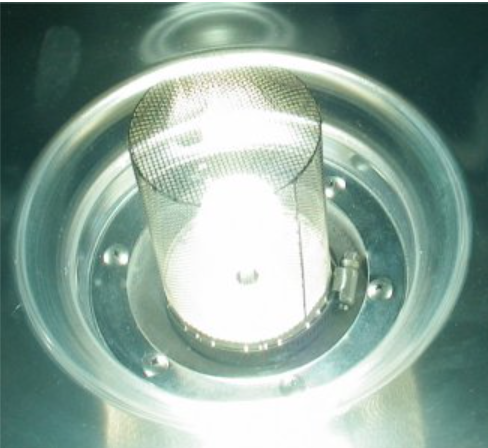
Accelerators
Accelerators, or ‘atom smashers’, accelerate charged particles (e.g. protons) to enormous speeds and energies and hurl them at other sub-atomic particles to learn about their constituent parts, and thus how all matter in the universe is constructed. In an electrostatic accelerator, the energy and velocity to which a particle is accelerated is determined by the voltage applied to output end of the accelerator. This voltage is limited by electrical breakdown. Alternatively, a wave propagating down a waveguide, designed so that the wave velocity is matched to the particle velocity, will continuously accelerate a particle without requiring super-high voltages. The charged particle can be thought of like a surfer riding on an ocean wave.

THz Imaging
Microwaves with mm wavelengths are used at airport security lines to scan for hidden weapons and explosives. The microwaves pass through the body, but different materials will absorb the waves differently. A shadow image is formed, which will reveal, for example, a concealed handgun. Unlike X-rays, THz radiation is non-ionizing and low power scanning has no harmful effects.
THz image reveals hidden weapon. (from https://sequestim.com/technology/– with permission)